Sounds of the Universe
Online Seminar with Prof. Richard de Grijs
The full video of the lecture is available here.
Does the Universe emit any sound? If yes, how are we able to listen to its chirps, blasts, roars, buzz, and pulsations? And how can we use this information?
These and many more questions were answered by Prof. Richard de Grijs, Astrophysicist and Associate Dean (Global Engagement) at Macquarie University, Sydney, Australia, during his online lecture on “Listening to the Universe” held on May 14, 2020.
In the context of the Understanding Science Seminars (US seminars), jointly organized by the UK Royal Society of Chemistry, Beijing University of Chemical Technology, the International Space Science Institute-Beijing (ISSI-BJ), as well as SELF (格致论道), this lecture represented the concerted effort to shorten the gap between science and the general public, i.e. science for the laymen, as well as to guarantee continuity to the US scientific talks despite the current unfortunate circumstances. The lecture was moderated by ISSI-BJ former Executive Director, Prof. Maurizio Falanga.
Thanks to Prof. de Grijs’ extensive experience and vast knowledge in the field of astrophysics and asteroseismology (or astroseismology), the audience was given the opportunity to explore and understand the Universe from an unusual point of view, i.e. its vibrations, its sounds, its music. In fact, despite it having large empty swathes and being usually considered an empty vacuum that does not allow matter propagation — in other words, sounds cannot be carried in space since sound waves need a medium to vibrate through — it is not completely empty, as it contains dust and gas left behind by old star and sometimes used to create new stars. Thus, this material represents the medium that makes it possible for sound waves to propagate in the Universe, even though at such a low frequency that they are beyond the capabilities of human hearing. However, with the right instruments, scientists are now able to detect the vibrations and signals in the Universe and convert them into sounds.
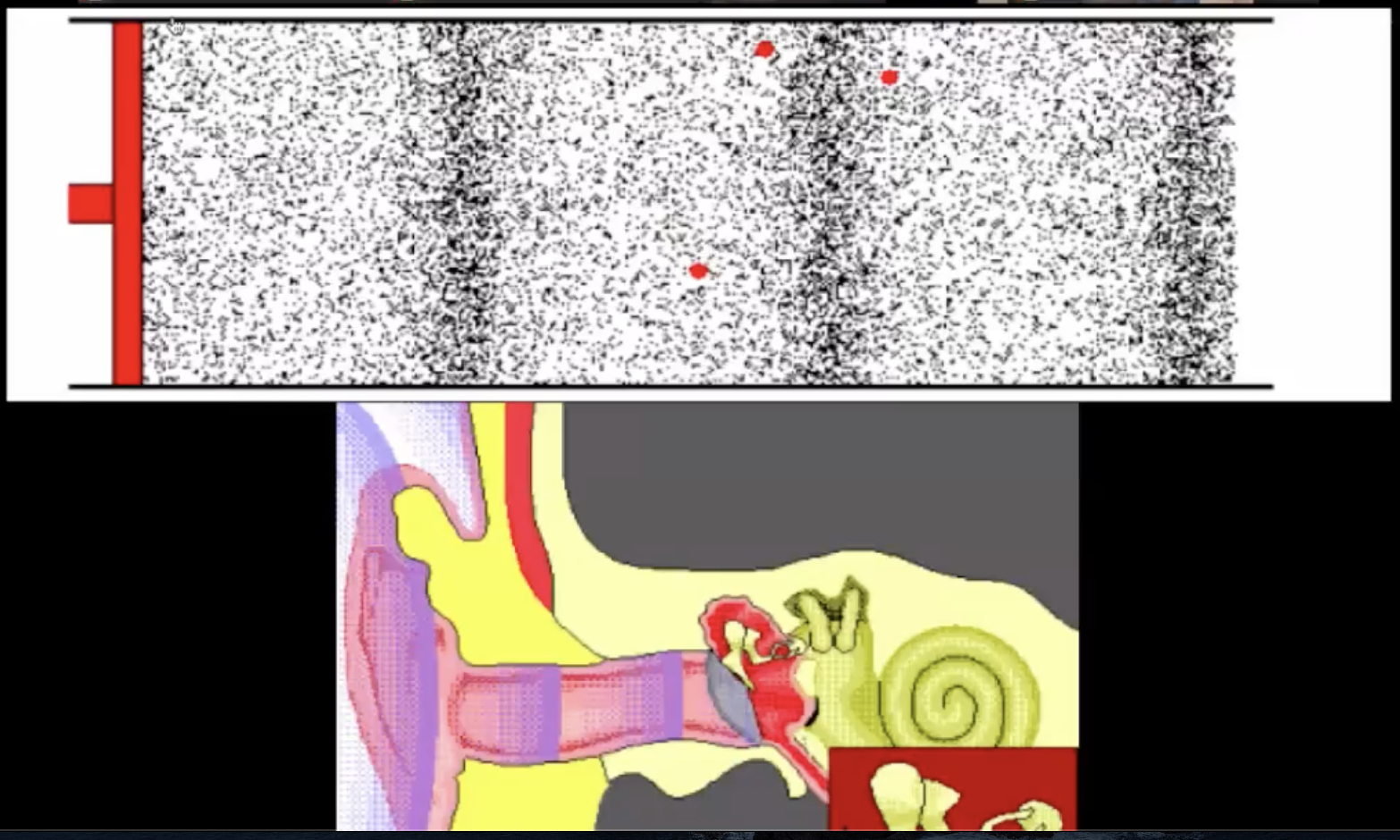
Figure 1 Sound waves — longitudinal waves, either vertical or horizontal — travel into the ear canal until they reach the eardrum. These vibrations are then passed by the eardrum through the middle ear bones into the inner ear and through the hearing nerve to the brain, where they are finally converted into sounds.
After a brief depiction of how hearing works and what sound waves are (see Figure 1), a short introduction to the sounds of the Earth was given. In fact, as earthquakes probably constitute the most representative sound emitted by our planet, the ‘noise’ produced by the massive Tohoku earthquake (Japan) in 2011 was played for the audience to get familiar with the sounds of the Earth. Following the voice of earthquakes, Prof. de Grijs moved to the sky and the near-Earth atmosphere by discussing the noises that can be derived from the Aurora Borealis and the Aurora Australis, respectively the northern and southern lights visible in high-latitude regions.
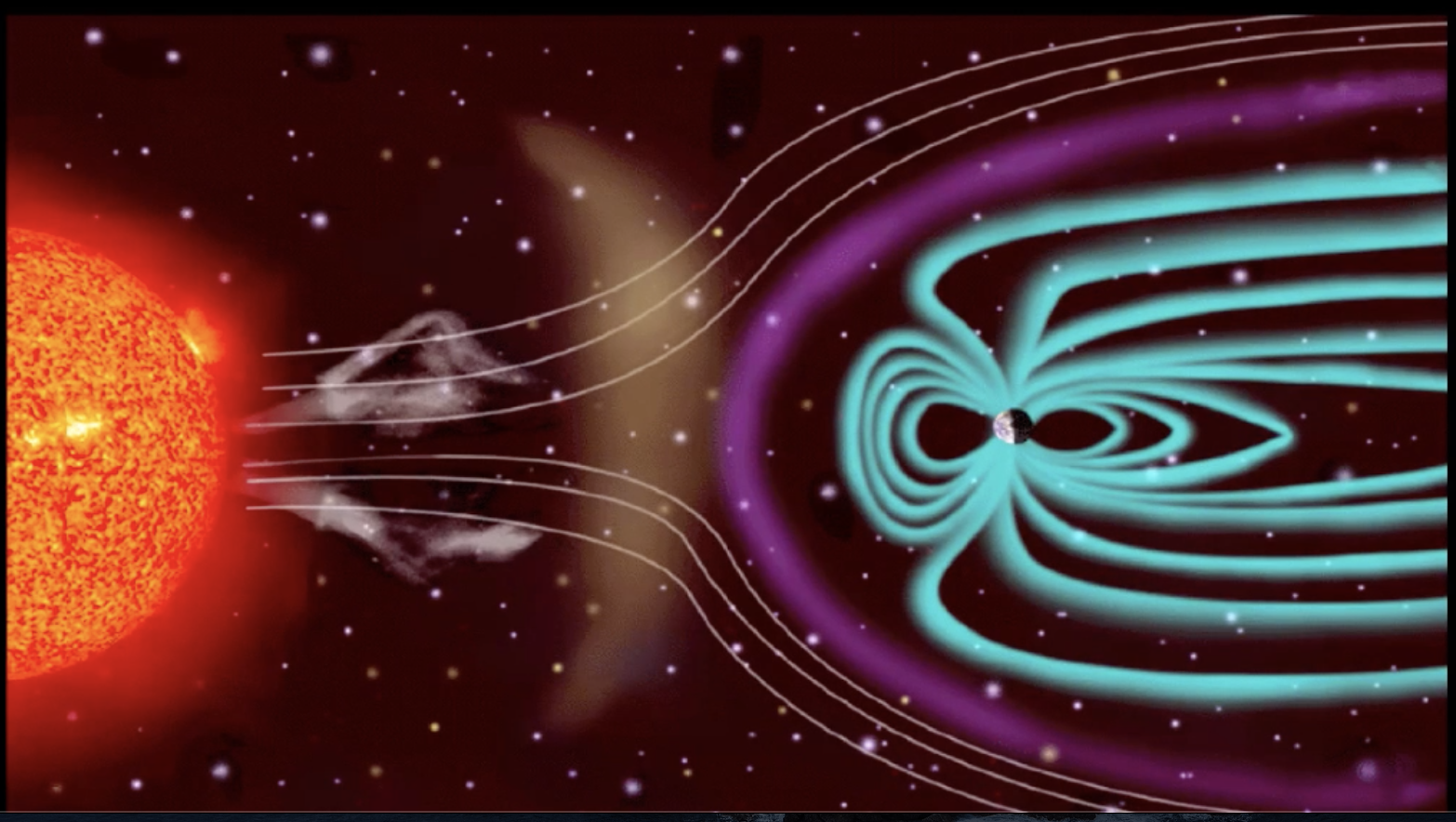
Figure 2 The Sun emits solar flares made of high-energy particles that hit the Earth's magnetic field, move around the heliosphere (purple screen) and interact with the Earth’s magnetic field. From this interaction, the Aurorae are thus produced and visible in high-latitude regions around the Arctic and Antarctic, especially in dark autumn and winter nights.
How are these Aurorae produced?
As the highly-charged particles emitted by the Sun and traveling at supersonic speed reach the protective outer layer of the Earth and hit the bow shock (see the yellowish bow in the center of Figure 2), they finally go around the magnetic field of the Earth (the blue lines connecting the north and the south pole) and hit the Earth near the Arctic and the Antarctic. The result of such interaction are the so-called northern and southern lights, which can then be converted into sounds.
Not only can these lights’ vibrations be heard, but also the Earth’s magnetic field can be transformed into hearable noise that can be used to measure the strength of the Earth’s magnetic field by means of a magnetometer, as achieved by the Bepicolombo European-Japanese satellite sent into space in 2018.
Figure 3 The BepiColombo joint mission satellite of the European Space Agency (ESA) and the Japan Aerospace Exploration Agency (JAXA) sent to the planet Mercury.
The lecture consequently moved up further from the Earth and to space, as Prof. de Grijs illustrated the sounds that we can be derived from the vibrations produced by the planets and stars present in our solar system. A graphic representation of the different stars’ 3D vibrations, their distribution, and speed, including the pulsation of red giants (bottom left in Figure 3) was provided. Such information about the frequencies of sound waves resonating inside of stars and caused by turbulence on the stars’ surface is very relevant for astronomers to better understand their internal structure, their age, and their mass.
Figure 4 The vibrations' pattern of stars.
Even further from our near-Earth space, stars with a much different vibration pattern were discovered in the 1960s by a Ph.D. student, Jocelyne Bell Burnell, a now very famous scientist and advocate of girls in sciences.
Since at first scientists had no idea what the origin of the very regular, heartbeat-like pulsations detected by Prof. Bell Burnell was — sent by the star CP1919 —, the signal was initially mistaken for a message coming from an alien civilization and therefore called LGM1 (standing for ‘Little Green Men’). Only later, thanks to a better understanding of the nature of these vibrations, this kind of celestial bodies were rebaptized Pulsating Stars, or Pulsars, as it was discovered that their pulsations are produced by a ray of light emitted by the star and hitting the Earth at a regular pace (see Figure 5). As explained by Prof. de Grijs, since Prof. Bell Burnell’s Ph.D. advisor Antony Hewish did not give her credit for this discovery, he was awarded the Nobel prize in Physics in 1974, thus stirring outrage in the scientific community.
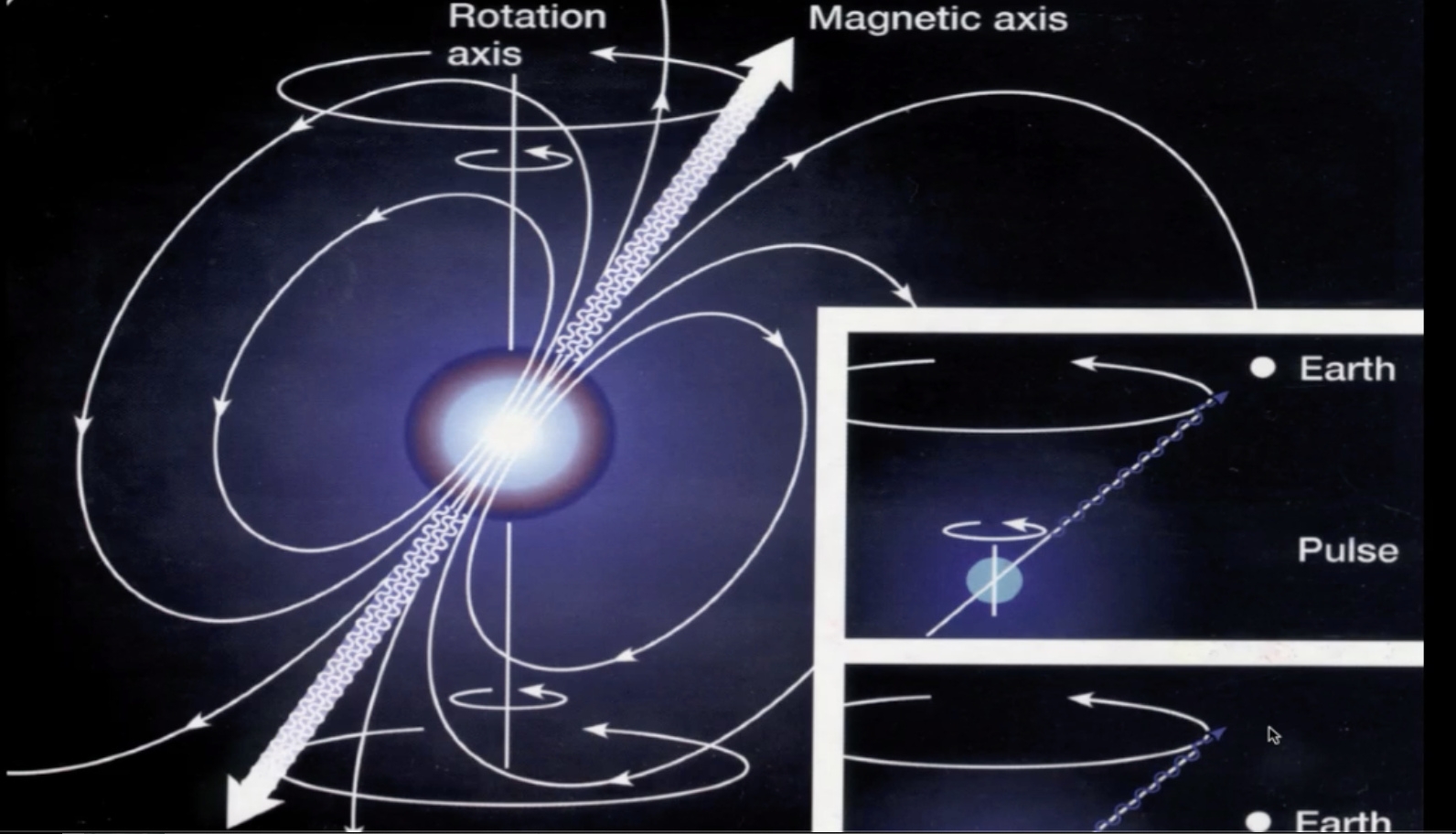
Figure 5 Pulsars can be better understood if compared to a lighthouse regularly hitting the Earth with its light beam. Since Pulsars’ magnetic axis is not aligned with the rotation axis of this kind of stars, their magnetic field funnels jets of particles out along the two magnetic poles, which conversely produce very powerful beams of light sweeping around as the star rotates.
Although these Pulsars have finally proved to be fast-pulsating stars and not alien signals, such discovery set the starting point for discussions on extraterrestrial civilizations, which consequently led to the establishment of the Search for Extraterrestrial Intelligence Institute (SETI) in California, USA, in the late 1960s. Since the very first conference on extraterrestrial intelligence held in 1969 — the Green Bank conference — did not have a specific agenda due to the lack of information and data on the topic, one of the SETI founders, Prof. Francis Drake, came up with a kind of equation — the so-called Drake Equation — to estimate the number of alien civilizations in the Milky Way galaxy.
Despite not having yet received any alien signal so far, scientists do every now and then pick up unknown signals coming from the Universe, such as the one from the Hercules galaxy cluster HD 156668, or the Wow! Signal (6EQUJ5), discovered in 1977 in Ohio, USA, and which, since it still remains unexplained, is deemed to bear the hallmarks of extraterrestrial origin, even though it happened only once.
Another kind of signal that scientists still do not fully understand is the so-called Fast Radio Burst, fast signals detectable all over the sky. Furthermore, given their scattered characteristic, scientists are wont to think that they are likely to be of extragalactic origin, i.e. signals coming from outside our Milky Way.
Figure 6 Two black holes orbiting around each other. The result of such interaction is what we call gravitational waves, while the merging sound of two black holes is called chirp.
Even though some of the signals detected by telescopes remain indecipherable, this should not necessarily lead us to jump to conclusions, meant Prof. de Grijs, as exemplified by the Peryton short radio signal detected by the Australian Parker telescope in 1998. Having initially left scientists puzzled and baffled about their origin, Perytons were later found to be the result of the premature opening of a microwave oven door located at the Parkes Observatory.
Moving further and further away from the Earth, Prof. de Grijs introduced the audience to the sounds detectable in galaxy clusters — i.e. groups of hundreds to thousands of galaxies — such as the Perseus galaxy cluster and produced by the gas-emitted X-rays. When a smaller galaxy cluster passes by, this is going to disturb the bigger galaxy cluster, and therefore, their interaction and merging process provokes disturbance waves, which are equivalent to sound waves. Moreover, massive galaxies contain (supermassive) black holes right in their center, which emit high-energy particles from their poles and keep bumping matter in the surrounding area, therefore producing longitudinal, sound waves.
Furthermore, when two black holes orbit around each other, before finally colliding and merging and thus producing a ‘chirp’ sound, they also produce gravitational waves, which expand through the Universe and reach the Earth, stretching and squeezing everything they pass through in an invisible way.
Finally, the conclusive image and sounds of the Universe provided by Prof. de Grijs consisted of the representation of our Universe as a function of time, from the Big Bang to the current galaxies’ pattern.
Figure 7 The Universe as a function of time. From left to right: The Big Bang, the first galaxies and black holes, normal galaxies, until the galaxies we can observe today in our local environment (far right).
The finding of the remnant of the Big Bang — the Cosmic Microwave Background (Figure 8) — earned the discoverers the Nobel Prize in 1978, and it represents the electromagnetic radiation from an early stage of the Universe, also known as "relic radiation". Observed with the European Planck Satellite, tiny temperature fluctuations were made visible in the background of the Universe, where the red-yellow spots are hot-temperature locations, while the blue ones are cold ones.
Figure 8 The Cosmic Microwave Background
As a conclusive remark, Prof. de Grijs actually played the sound of the expansion of the Big Bang, i.e. of the Cosmic Microwave Background.
The lecture was concluded with some questions taken online and moderated by Prof. Falanga.
----